Unsteady Flow Examples
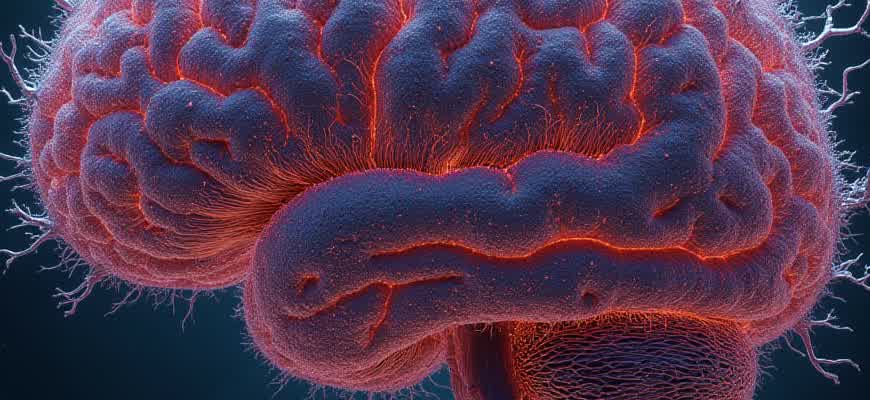
Non-steady fluid motion refers to the type of flow where the velocity at any given point changes over time. Several common instances of such flow can be observed in various practical and natural systems.
Some typical cases of unsteady flow include:
- Transient Flow in Pipes: When a valve is suddenly opened or closed, the flow rate and pressure within the pipe change over time.
- Flow Around an Object: The airflow over a moving vehicle or an aircraft changes dynamically as speed varies.
- Water in Open Channels: The flow in rivers and streams often experiences variations due to rainfall, dam releases, and other external factors.
In the following table, we summarize the key characteristics of these unsteady flow examples:
Flow Type | Cause of Variability | Typical Scenario |
---|---|---|
Pipe Flow | Sudden changes in valve settings | Opening or closing a valve |
Flow Around Objects | Movement of the object or variation in external conditions | Aircraft or car motion |
Open Channel Flow | Environmental factors like rainfall or water release from reservoirs | River or stream dynamics |
Non-steady flow is characterized by fluctuations that occur over time, leading to temporary changes in the velocity and pressure within the flow field.
Analyzing the Role of Unsteady Flow in Fluid Dynamics Simulations
Unsteady flow plays a crucial role in accurately modeling real-world fluid behavior, especially when the velocity, pressure, or other flow parameters vary with time. These dynamic variations can be caused by external forces, system geometry changes, or boundary conditions that evolve over time. In fluid dynamics simulations, capturing the transient nature of these flows allows for more realistic predictions and optimization of systems such as engines, HVAC, and aerodynamics.
The primary challenge in simulating unsteady flow is the need for higher computational power and more complex mathematical models. Unlike steady-state simulations, where the flow is assumed to be constant over time, unsteady flow requires time-dependent solutions to governing equations like the Navier-Stokes equations. This leads to intricate numerical methods that can accurately capture temporal changes in the flow fields.
Key Considerations in Unsteady Flow Simulations
- Time Resolution: Accurate representation of unsteady flow requires fine time steps to capture rapid changes in velocity and pressure.
- Nonlinearity: Many unsteady flows, particularly turbulent ones, are governed by nonlinear behavior, which introduces additional complexity in simulations.
- Boundary Conditions: Variations in boundary conditions over time significantly impact the flow dynamics and must be modeled with precision.
The accuracy of unsteady flow simulations heavily depends on the temporal resolution of the model. Insufficient time step sizes can lead to inaccuracies in predicting flow characteristics.
Applications of Unsteady Flow Simulations
- Aerospace Engineering: Simulations of unsteady flows help in designing efficient aircraft and understanding the effects of turbulent wakes, vortex shedding, and shockwaves.
- Automotive Engineering: In engine simulations, transient flow analysis allows for optimizing fuel injection, combustion processes, and exhaust systems.
- Environmental Engineering: Predicting unsteady flows in natural systems, such as river currents or atmospheric flows, helps in managing water resources and predicting weather patterns.
Comparison of Unsteady vs. Steady Flow Simulations
Aspect | Unsteady Flow | Steady Flow |
---|---|---|
Time Dependency | Time-varying | Constant over time |
Computational Complexity | High, requires finer time resolution | Low, simpler models |
Application | Dynamic systems with time-varying conditions | Systems with constant flow conditions |
Implementing Unsteady Flow Concepts in Real-World Engineering Projects
Unsteady flow behavior is crucial to understand in various engineering applications, including hydraulic systems, aerodynamics, and thermal systems. Engineers need to incorporate these principles to predict performance accurately and optimize system design. Implementation involves analyzing fluctuating pressures, velocities, and other parameters that vary with time. Proper modeling and simulation are key components to achieve realistic results in these dynamic systems.
When applying unsteady flow concepts, engineers must adopt a systematic approach that integrates both theoretical and computational tools. Computational Fluid Dynamics (CFD) is commonly used to simulate transient behavior, while experimental testing may also provide valuable insights. The following steps highlight the general process for implementing these concepts in engineering projects:
Steps for Implementing Unsteady Flow in Engineering Projects
- Define System Requirements: Identify the transient behavior, such as sudden changes in flow or varying operating conditions.
- Select Appropriate Models: Choose suitable mathematical models and numerical methods that can capture the time-dependent nature of the flow.
- Perform Simulations: Use CFD tools or other simulation software to predict how the system responds to changes in parameters.
- Validate Results: Conduct experimental tests to verify the accuracy of the predictions and refine the model if necessary.
- Optimize Design: Make adjustments based on simulation results to improve system efficiency and reliability.
Key Considerations: Unsteady flow analysis requires attention to time scales, turbulence models, and boundary conditions that vary dynamically over time. Understanding the specific characteristics of the flow is essential for selecting the right simulation tools and accurately interpreting results.
"Unsteady flow is not only a theoretical challenge but a practical necessity for optimizing engineering systems under real-world conditions."
Real-World Example: Pump Systems
In pump systems, unsteady flow can be observed when there are sudden changes in the flow rate due to valve operations, pressure fluctuations, or changes in power input. This can lead to phenomena like water hammer, which may damage pipes and valves. To manage such conditions, engineers must:
- Perform transient simulations to understand pressure peaks.
- Design surge tanks or other protective devices to mitigate flow-induced stresses.
- Use monitoring systems to detect any unexpected changes in flow patterns.
Phase | Action | Tool/Technique |
---|---|---|
Initial Design | Model flow variations | CFD simulation |
Testing | Validate results | Experimental testing |
Optimization | Modify system parameters | Dynamic adjustments |
Understanding the Mathematical Models Behind Unsteady Flow Patterns
Unsteady flow refers to fluid motion where velocity or pressure changes with time. This dynamic behavior is crucial in various applications, from weather systems to engineering systems like pumps and turbines. The mathematical models used to describe unsteady flow rely on complex equations that capture these time-varying changes. These models help predict how fluids behave under different conditions, allowing engineers and scientists to design more efficient systems and understand natural phenomena better.
The core principle behind unsteady flow analysis is the conservation of mass, momentum, and energy. These principles form the foundation of the governing equations, including the Navier-Stokes equations. The challenge in unsteady flow is solving these equations for specific boundary conditions and initial conditions, as the solutions often involve time-dependent variables. Let's explore some common mathematical approaches used in the study of unsteady flows.
Key Mathematical Concepts in Unsteady Flow
The mathematical representation of unsteady flow involves several key components:
- Navier-Stokes Equations: These are the fundamental equations governing fluid motion and are used to describe both steady and unsteady flows.
- Continuity Equation: This equation ensures the conservation of mass, representing the relationship between the fluid density, velocity, and pressure over time.
- Energy Equation: This equation accounts for the conservation of energy within the fluid system, including internal energy and heat exchange effects.
Note: Solving these equations in unsteady flow often requires numerical methods, especially for complex geometries or turbulent flows, where analytical solutions are not feasible.
Approaches for Solving Unsteady Flow Equations
There are different methods to solve the governing equations of unsteady flow, depending on the complexity of the flow. Here are some common approaches:
- Finite Difference Method (FDM): This method involves discretizing the time and space domains and solving the resulting system of equations iteratively.
- Finite Volume Method (FVM): This technique focuses on conservation laws and is widely used for computational fluid dynamics (CFD) simulations.
- Spectral Methods: These methods decompose the solution into basis functions and are useful for high-precision simulations of fluid flow in simple geometries.
Common Applications
Unsteady flow models are applied in various domains such as:
Application | Example |
---|---|
Pump Design | Modeling transient behaviors in pump systems to avoid cavitation and enhance performance. |
Weather Prediction | Simulating airflow in the atmosphere to predict storm patterns and air circulation. |
Aerospace Engineering | Studying unsteady airflow over wings and fuselages during different flight conditions. |
Tools and Software for Simulating Unsteady Flow in Various Environments
Simulating unsteady flow requires specialized software tools capable of capturing complex fluid dynamics over time. These tools are particularly valuable in fields such as aerodynamics, hydrodynamics, and civil engineering, where the flow is not constant and exhibits time-varying behavior. Such simulations are essential for understanding transient events, like turbulent fluid motion, shockwave propagation, or sudden changes in environmental conditions.
Numerous software packages are available for modeling unsteady flow. These tools leverage numerical methods to solve the governing equations of fluid dynamics, such as the Navier-Stokes equations, and incorporate time-dependent changes in velocity, pressure, and other physical quantities. Below is a list of popular tools and their features.
Popular Simulation Tools
- ANSYS Fluent: A widely used computational fluid dynamics (CFD) software that can handle both steady and unsteady flows. It is capable of simulating complex transient phenomena in various environments.
- OpenFOAM: An open-source CFD toolkit known for its flexibility and extensive libraries, particularly suited for time-dependent flow simulations in research and industry applications.
- COMSOL Multiphysics: A multi-physics simulation software that integrates unsteady flow analysis with other physical domains, such as heat transfer and structural mechanics, making it versatile for multiphysics problems.
- SimFlow: A more accessible CFD software designed for both novice and advanced users, providing robust unsteady flow simulation capabilities with a user-friendly interface.
Key Features of Simulation Tools
Software | Key Features |
---|---|
ANSYS Fluent | Advanced turbulence models, transient flow simulations, detailed post-processing capabilities. |
OpenFOAM | Highly customizable, large library of solvers, parallel computation support. |
COMSOL Multiphysics | Multi-physics coupling, specialized for coupling flow with structural, thermal, and electrical analysis. |
SimFlow | Simple interface, high-quality results, and support for both steady and transient analysis. |
Note: It is important to choose the right simulation tool based on the complexity of the flow scenario and the available computational resources.
Choosing the Right Tool for Your Application
- Assess the complexity of the flow you intend to simulate. Tools like OpenFOAM are better suited for research or highly customized applications, while ANSYS Fluent offers a more comprehensive, user-friendly approach for commercial projects.
- Consider the computational resources available. Some tools, like OpenFOAM, require high computational power for large-scale simulations, while others, like SimFlow, are optimized for less intensive computing environments.
- Examine the specific features needed. If your simulation involves multi-physics interactions, COMSOL Multiphysics may be the most appropriate choice due to its versatile nature.
Practical Applications of Unsteady Flow in Environmental and Coastal Engineering
In environmental and coastal engineering, the analysis of unsteady flow is essential for understanding various dynamic processes. Unsteady flow refers to the variations in flow parameters such as velocity and pressure over time, which is commonly observed in natural systems. Engineering applications involving rivers, estuaries, coastal areas, and stormwater systems heavily rely on this concept. The primary challenge in these environments is the need to account for the unpredictable changes caused by tides, storms, and human interventions.
Among the most critical uses of unsteady flow analysis are flood management, sediment transport studies, and the protection of coastal structures. It is essential to evaluate how transient forces affect the behavior of water bodies to design appropriate mitigation strategies. For example, unsteady flow modeling helps in predicting the movement of pollutants in estuaries or understanding how waves and currents influence the erosion of coastal landforms.
Key Applications in Coastal and Environmental Engineering
- Flood Risk Assessment: Unsteady flow modeling is vital for understanding flood dynamics during heavy rainfall or storm surges.
- Sediment Transport: Coastal engineers use unsteady flow analysis to predict how sediment moves along shorelines under varying tidal conditions.
- Stormwater Management: Stormwater systems rely on unsteady flow models to optimize drainage designs and reduce the risk of urban flooding.
Table: Examples of Unsteady Flow Models in Coastal Engineering
Application | Model Used | Purpose |
---|---|---|
Floodplain Mapping | Hydrodynamic Models (e.g., MIKE 21) | Simulate flood propagation and assess flood risks during storm events. |
Sediment Transport | SHORECIRC Model | Estimate sediment movement due to unsteady tidal currents and wave action. |
Coastal Erosion | SWAN Model | Simulate wave-driven sediment transport and the erosion of coastal structures. |
“Understanding unsteady flow is crucial for developing sustainable solutions in coastal engineering, especially when dealing with complex tidal and storm surge interactions.”
Challenges When Dealing with Unsteady Flow and Strategies for Overcoming Them
Unsteady flow, or time-varying flow, presents several challenges in fluid mechanics, as it involves continuous changes in flow velocity and pressure within a system. Engineers often encounter difficulties in predicting the behavior of the flow, understanding transient dynamics, and implementing accurate models. The inherent complexity of unsteady flow can lead to errors in design, inefficient performance, or even system failure if not handled properly.
Addressing these challenges requires both theoretical knowledge and practical expertise in flow modeling, computational techniques, and system control. Below are some of the common obstacles faced when working with unsteady flows and the strategies used to mitigate them.
Common Challenges
- Complexity of Fluid Behavior: Unsteady flows exhibit nonlinear behaviors, making it difficult to predict flow patterns over time.
- Time-Dependent Boundary Conditions: Varying conditions at the boundaries complicate the solution of governing equations, requiring adaptive models.
- Inadequate Computational Models: Many flow simulations fail to capture transient phenomena accurately, leading to unreliable predictions.
Strategies for Overcoming Challenges
- Use of Advanced Computational Methods: Implementing Computational Fluid Dynamics (CFD) with transient solvers can provide more accurate results for unsteady flow systems.
- Implement Adaptive Mesh Refinement: Increasing the resolution of the computational mesh during transient periods can improve the accuracy of simulations without excessively increasing computational cost.
- Robust Control Systems: Using real-time feedback and control systems can help in adjusting to transient changes and stabilizing the flow.
Tip: Careful calibration of numerical models and ongoing validation with experimental data are key to improving the accuracy of predictions in unsteady flow simulations.
Example of Unsteady Flow Challenge
Scenario | Challenge | Solution |
---|---|---|
Pipes with pulsating flow | Unpredictable pressure and velocity variations | Use of dynamic boundary conditions and real-time flow monitoring systems |
Flow over an oscillating surface | Difficulty in simulating accurate boundary layer development | Refinement of mesh and time-stepping algorithms |